Blog
Ultrafast dynamics of transition metal dichalcogenide
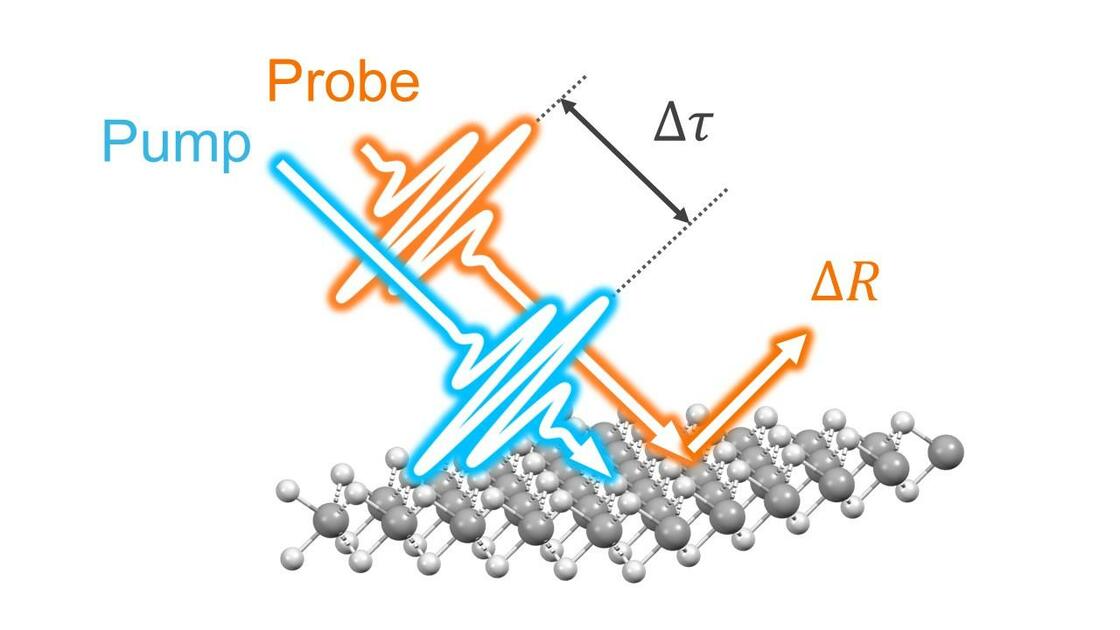
Physical Properties of Transition Metal Dichalcogenides
Transition Metal Dichalcogenides (TMDC) are crystals that consist of a single transition metal layer sandwiched between layers of chalcogen atoms (see Transition Metal Dichalcogenides article for details). As shown in Figure 1, a single-layer TMDC has a thickness of only three atoms and exhibits strong quantum confinement effects in out-of-plane direction (perpendicular to crystal plane). In TMDCs with semiconductor properties, electron-hole pairs (excitons) are formed inside when excited, but electron-hole distance (Bohr radius) shrinks due to quantum confinement effect in out-of-plane direction. Electron-hole distance (Bohr radius) shrinks due to quantum confinement effect in face direction. Since there is little screening effect against electron-hole Coulomb interactions from other atoms, exciton binding energy is very large, about 550 meV. Therefore, exciton can exist stably even at room temperature.
Fig. 1: Crystal structure of single-layer TMDC
Ultrafast Dynamics
Since carrier excitation and relaxation processes in semiconductors occur in ultrashort time region of femtosecond to picosecond scale, pump-probe measurement using an ultrashort pulse light source can elucidate exciton polariton relaxation path and relaxation dynamics. In pump-probe measurements, two femtosecond pulses, a pump light and a probe light, are injected into sample. Pump light forms exciton polaritons in the sample, and change in refractive index is monitored by reflectance of probe light. By delaying timing of probe light incidence, generation and relaxation of exciton polariton can be followed like an animation.
In example shown below, a single-layer MoSe2 sample grown by chemical vapor deposition (CVD) on a silicon substrate was used to investigate its dynamics by pump-probe measurements. Measurements were performed in a room temperature environment, and a pump light of 400 nm, which is much higher than band gap (band gap width of MoSe2 is 800 nm), was used for pump light. Probe light was 800 nm light with exciton absorption. Schematic diagram of pump probe measurement, data and fitting results are shown in Figure 2.
Fig. 2: Ultrafast dynamics of monolayer MoSe2
Following function was used for fitting.
$$y = y_0 + A\exp\Big(-\frac{t}{\tau_f}\Big) + B\exp\Big(-\frac{t}{\tau_s}\Big)$$
Relaxation Process
When MoSe2 is irradiated by pump light with energy much greater than band gap, electrons are excited and carriers are formed in conduction band. Therefore, relaxation to the bottom occurs first inside conduction band, followed by relaxation to excitonic state. Thereafter, carrier recombination occurs through two relaxation processes: radiative and non-radiative recombination.
Radiation Processes
It has been reported that interband transitions dominate dynamics in radiation process [1]. When pump light is incident, the excited carriers are localized much higher than the band gap and, in other words, have arbitrary wavenumbers. Across thermal relaxation to phonons, etc., the wavenumber of carriers takes the same value as that of the upper end of the valence band, and they are recombined through a luminescence process by photon emission. This process is the main contributor to the luminescence from the monolayer. In a previous study, the luminescence lifetime of monolayer MoSe2 was measured at cryogenic temperatures (7 K) and was reported to be about 1.8 ps.[2] It is reported that the properties of MoSe2 and MoS2 change from indirect transition type semiconductors in the bulk sample to direct transition type semiconductors with high luminescence efficiency in the monolayer sample. Figure 3 shows the transition of the band structure as the number of layers changes.
Fig. 3: Transition of the band structure of MoS2 [3]
Luminescence processes occur at the $K$ point of Brillouin zone, but in the bulk sample, lower end of the conduction band is at $\Gamma$ point. Therefore, the lower end of the conduction band moves away from the $K$ point as the number of layers increases, and higher-order processes such as scattering by phonons that incorporate the difference in wavenumber vectors are required for interband transitions to occur. Thereby, the relaxation paths change.
Non-radiative Processes
In non-radiative recombination processes, excitons with strong exciton binding energy stay in the crystal for a while after excitation. In previous studies, exciton-exciton annihilation (EEA), such as the Auger process, is dominant in the region of 3-8 ps after excitation. Since EEA is proportional to the square of the exciton density, the number of excitons produced by increasing the power of the pump light is proportional to the power of the pump light. As the EEA is proportional to the square of the exciton density, the time required for the EEA relaxation process ($\tau_f$) becomes shorter as the pump light power increases and the number of excitons produced increases[3], which has a larger effect than in a linear process. At 30-80 ps, the dominant process is carrier-phonon scattering. In this process, excitons are trapped by defects in the crystal and become bound excitons, and the energy difference is emitted as phonons (heat and lattice vibrations). The more defects in the crystal, the faster the exciton is trapped, and the shorter the time ($\tau_s$) for this relaxation process [4].
Formation of Trion and biexciton
As mentioned above, excitons formed in TMDCs have very strong binding energy, so there are particles with special structures. These are particles called trions, in which an electron or hole is bound to an electron-hole pair, and biexcitons, in which another electron-hole pair is bound to an electron-hole pair. Figure 4 shows a schematic of trion and biexciton.
Fig. 4: Trion, biexciton
Trions have a positive or negative charge and are very unstable under normal conditions. However, excitons generated in two-dimensional materials such as TMDC have very strong binding energy, so that even particles with such an unstable structure can exist stably at room temperature. The extra charge is generated from defects in the substrate and other sources [5]. Each particle has a different binding energy from exciton, with trion being 30-31 meV and biexciton being about 20 meV in MoSe2. In a previous study, it was reported that the time constant for exciton relaxation in MoS2 is 1.2 ps, whereas the relaxation of electron 2 is very slow, 56.6 ps. This is because the exciton is formed at 500-600 fs after pumping and then the electron is trapped to become a negatively charged trion (negative trion) [5].
References
[1] Robert C. et al. Exciton radiative lifetime in transition metal dichalcogenide monolayers. Phys. Rev. B, 2016, 93(20), 205423
[2] Liu H. et al. Exciton Radiative Recombination Dynamics and Nonradiative Energy Transfer in Two-Dimensional Transition-Metal Dichalcogenides, J. Phys. Chem. C, 2019, 123(15), 10087-10093
[3] Splendiani. A. et al. Emerging Photoluminescence in Monolayer MoS2. Nano Lett. 2010, 10(4), 1271-1275
[4] Yuan L. et al. Exciton Dynamics, Transport, and Annihilation
in Atomically Thin Two-Dimensional Semiconductors, J. Phys. Chem. Lett., 2017, 8(14), 3371–3379.
[5] Tanmay G., et al., Ultrafast Carrier Dynamics of the Exciton and Trion in MoS2 Monolayers Followed by Dissociation Dynamics in Au@MoS2 2D Heterointerfaces, J. Phys.